The New Net Zero

Achieving Greenhouse Gas Mitigation Targets through Life Cycle Carbon Accounting
Climate change is the existential crisis of our time. The design and construction industry has responded to this challenge with a wave of green buildings that have reshaped expectations for environmental performance of the built environment, striving to meet increasingly stringent energy codes, rating systems, and greenhouse gas reduction targets. However, we are only solving the problems that we are looking at, and we are not seeing the whole picture. The substantial up-front carbon emissions associated with the production of building materials and construction have gone largely uncounted, as have those associated with demolition—but they are no less real and just as significant. New, high-performance buildings are designed to reduce emissions over the life of a building, but when will that payoff occur? Thirty years from construction? Fifty? Unfortunately, we cannot wait fifty or even twenty years for our new, efficient buildings to save us. Design and construction practices must be dramatically and immediately reshaped to drive down emissions associated with all stages of a building’s life—including materials, construction, and demolition—in order to meet critical global climate goals.
The Urgent Need to Reduce the Life Cycle Carbon Footprint of Buildings
The 2015 Paris Climate Agreement established the necessity of capping global temperature rise to well below 2° Celsius, setting the target at 1.5°. In order to achieve that target, the world needs to get to net zero carbon emissions by the year 2050. According to the 2018 UN Environment Emissions Gap Report, we are not on track to meet this goal [i]. In fact, we must now reduce global emission by 50% by the year 2030 to have even a 50% chance of meeting the goals of the Paris Climate Agreement [ii].
At a global scale, building construction and operations account for approximately 39% of carbon dioxide emissions annually [iii]. We are also projected to build an astounding 2.5 trillion square feet of new construction globally by the year 2060 [iv], roughly doubling the current square footage of existing buildings. This is an extraordinary figure, and it means that building owners and professionals in the construction sector have a substantial opportunity and responsibility to reduce building-related carbon emissions to respond to the climate crisis.

The paradigm of sustainable design has for decades focused on reducing operational energy, with ultimate goals of net zero or even net positive carbon. This approach has driven energy reduction goals in codes, rating systems, and even carbon mitigation plans. While reducing operational carbon is a critical component to driving down carbon emissions in the built environment, “net zero” used in this context is a misnomer. The definition of net zero in the United States only includes the carbon emissions associated with the use phase of the building, also known as operational carbon, while excluding emissions associated with all other stages of a building’s life cycle, such as up-front carbon emitted during the production of materials and building construction, also referred to as embodied carbon, and emissions associated with end-of-life.

Image by: Stacy Smedley/Skanska
While we have made strides in reducing the operational energy of buildings, with an average global reduction of 1.5% annually [v], the impacts of embodied carbon have been overlooked. These embodied impacts were for many years thought to be negligible; however, it has become increasingly apparent that they are not insignificant. Embodied carbon contributes at least 11% of all global carbon emissions annually—and this percentage is increasing. In fact, projections made by the nonprofit research organization Architecture 2030 show that by the year 2030, embodied emissions will account for 74% of total emissions from all buildings constructed after 2020. As buildings meet higher performance standards and the grid becomes greener, operational carbon will continue to decrease, and embodied carbon will represent an increasingly large percentage of total life cycle emissions.
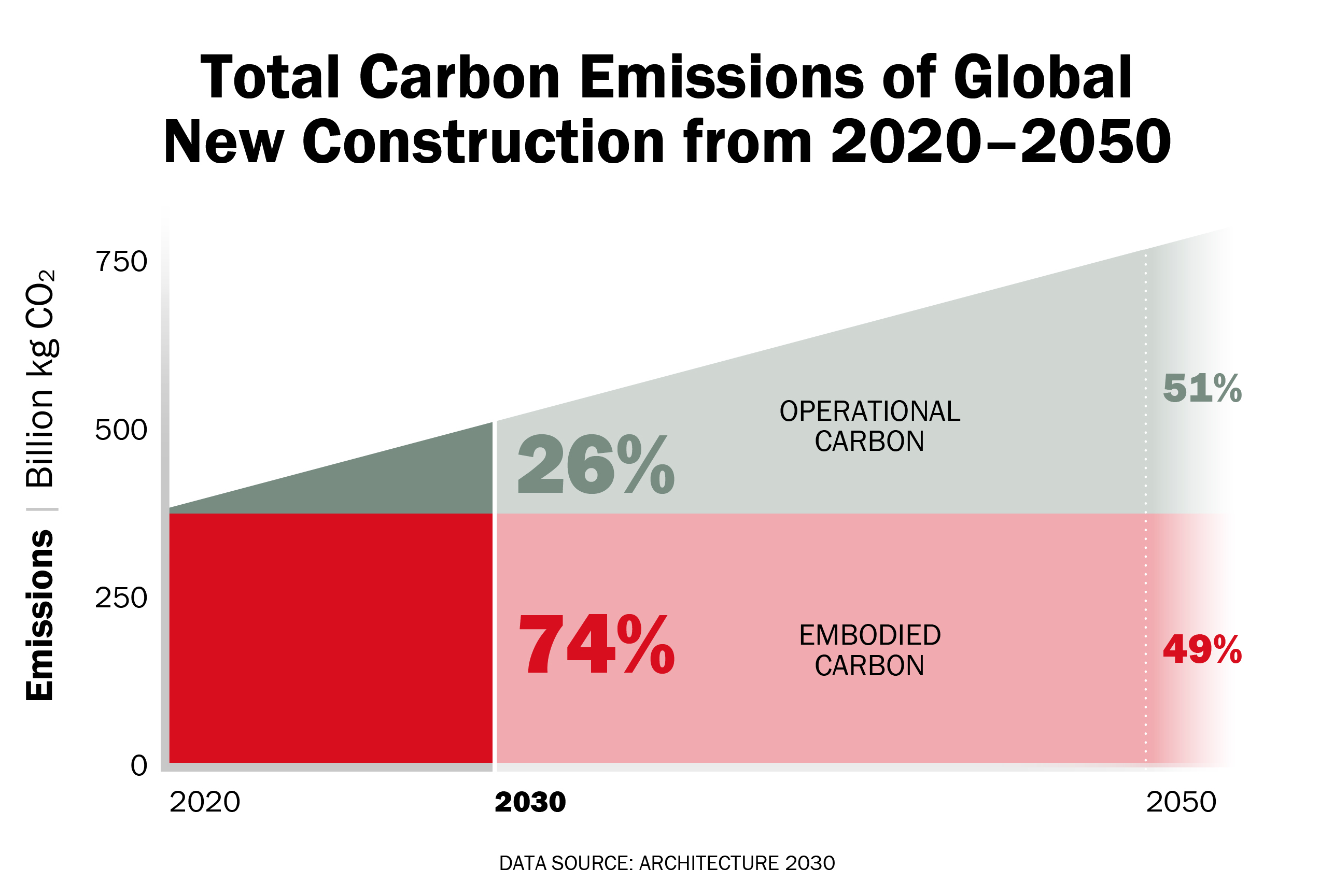
Because sustainable design practices have responded to what our industry has measured to-date—operational carbon—our industry has primarily focused on new, high-performance construction. This has reinforced the misconception that new buildings are good for the environment while existing buildings are energy hogs that should be demolished and replaced. However, the fact that the majority of carbon emissions associated with the built environment are operational emissions from existing buildings means that renovations are one of our biggest opportunities to reduce building-related environmental impacts. Since reusing an existing building inherently results in lower embodied emissions, operational emissions reductions in these buildings can be relatively more impactful in the near-term than is true for new construction.
When total carbon emissions are evaluated over a building’s full life cycle and within the context of 2030, or even 2050, as the critical point of no return, it becomes clear that our climate goals are not achievable through operational carbon reductions alone. Owners, designers, contractors, and manufacturers need to radically re-imagine the way we use, renovate, and design buildings, and we need to do so with a sense of urgency. This collaborative transformation is underway. The data and tools needed to quantify life cycle environmental impacts of the built environment are being developed and refined, and the strategies to reduce these impacts through new materials, smarter design, and building reuse exist and are quickly advancing.
Quantifying Embodied Carbon and Avoided Impacts
Tracking the environmental impacts of the full building life cycle from material extraction through demolition is essential to achieving carbon neutrality within the necessary time-frame. Carbon accounting, broadly speaking, is the practice of quantifying the carbon dioxide emitted into the atmosphere from any entity or system. In the case of buildings, this translates to the carbon emissions associated with building materials, construction activities, building use and maintenance, and demolition. Each building has its own carbon profile, which begins with a certain amount of up-front emissions from construction, grows with use over time, and has intermittent spikes at points of renovation and component replacement. These impacts are measured and analyzed through a process called life cycle assessment (LCA).
Life cycle assessment is a four-step process that consists of:
- Defining the scope and boundaries of the assessment (i.e., a material, assembly, or building)
- Creating an inventory of all the components within that scope (i.e., a bill of materials)
- Assessing the environmental impacts, which are calculated by multiplying each material quantity by a series of environmental impact factors
- Interpreting the results, which are the environmental impact of the subject measured across a series of impact categories, including global warming potential, acidification potential, and smog formation potential.
LCA methodology can be applied to a single material, such as paint; an assembly, such as a window; or to a whole building. If you are analyzing a single window, the inventory of materials is relatively small. If you evaluate an entire building, you may have hundreds of materials to consider.
Over the past several decades, energy modeling to predict the operation energy use of buildings has become a standard part of design practice. Embodied carbon can similarly be determined using life cycle assessment data and tools. These tools take many forms, from Environmental Product Declarations
(EPDs) to Whole Building Life Cycle Assessment (WBLCA) software. Manufacturer EPDs are increasingly available, providing transparency for individual materials specified on a project. WBLCA software, including stand-alone software from Athena and Revit-integrated tools like Tally and One-Click, allows designers to predict the environmental impacts of their decisions throughout the design process. Tools such as the recently released Embodied Carbon in Construction Calculator (EC3) are assisting with estimated embodied carbon emissions for projects while also encouraging manufacturers to disclose and drive down the footprint of their products.
Integrating energy model results into a life cycle assessment provides a complete view of life cycle emissions. The integration of embodied and operational impacts enables the examination of the true benefits of design upgrades associated with high performance. For example, how much embodied carbon is associated with adding an extra inch of insulation to the walls and roof of a building? What about triple-glazed versus double-glazed windows? How much do those choices reduce the operational carbon emissions? What is the payback? Is it before the year 2050? This big picture framing provides the opportunity to make meaningful reductions in carbon emissions during the timeframe when they matter most.
Embodied Carbon Reduction Strategies
There is no single solution that will fully achieve the emissions reductions required from our built environment. We need strategies that work for all scales of construction, from single-family houses to high-rise towers. We need to address existing buildings of all ages in addition to new construction. And we need to reduce carbon emissions associated with all stages of a building’s life cycle. Two nearly universal strategies for reducing up-front, embodied emissions are critical to meeting our immediate carbon reduction targets: 1) use better materials, and 2) use less material.
Before implementing either strategy, it is important to understand which materials have the highest environmental impacts, and thus provide the greatest opportunity for improvement; this can be accomplished with LCA or using general rules of thumb. The materials with the greatest impact are typically the materials that occur in the greatest quantity in a building; for a renovation that may be drywall and steel studs, while for new construction it is typically structure and envelope.
The first embodied carbon reduction strategy is to use better materials. Although there are many important factors designers should consider, such as impacts to human health and social equity in the supply chain, “better materials” here means materials with a lower carbon footprint. Concrete is a prime example of a building material with a large carbon footprint that is present in nearly every construction project. Cement, the primary ingredient in concrete, accounts for between 6% and 10% of all global carbon dioxide emissions [vi]. A study performed for the City of Vancouver calculated that simply increasing the substitute cementitious material in a building’s structure from 10% to 35% reduced the embodied carbon of the building by 22.6% [vii].
While improving a concrete mix is an example of substantially improving a high-intensity material, there are also huge opportunities in substituting plant-based, carbon-storing materials for their traditional, high-intensity counterparts. There is an increasing range of building products made from biogenic materials such as wood members, cork or wool insulation, and strawboard wall panels that store rather than emit carbon dioxide. A recent study of single family residences found that a best-case design using biogenic materials could provide net carbon storage for several hundred years of operation [viii]. By rethinking our approach to building materials and life cycle carbon, the built environment can become an asset in combatting climate change rather than a liability.
The second strategy for reducing embodied carbon is to use less material. This is where building reuse emerges as a critical path towards meeting carbon reduction targets. Reuse of existing building fabric can range in scale from using salvaged materials up to whole-building renovation. Since we know that structure and enclosure generally represent the largest percentage of embodied impacts in a building, reusing these components is the most impactful way to reduce embodied emissions. Case studies of LCA applied to comprehensive renovation projects show that reusing an existing building results in embodied emissions reductions ranging from 50% to 85% when compared to new construction; the more fabric that is reused (and the less that is added back), the greater this percentage reduction. Through the judicious use of new materials and systems in conjunction with reuse of primary structure and facade elements, renovations can result in dramatically reduced emissions across all use stages by minimizing embodied carbon, greatly reducing operational carbon, and eliminating the majority of end-of-life demolition waste. When evaluated through the year 2050, renovation is consistently shown to have lower total carbon emissions compared to demolition and new construction [ix, x].
New construction offers its own opportunities for reductions in material quantity. Reducing a building’s square footage is one way to use less material; designs that maximize spatial and plan efficiency reduce both the mass of structure and the area of enclosure. Another method is to optimize the efficiency of a building’s structural system by designing with spans and configurations appropriate to the structural material. This can measurably reduce the quantity of structural material without sacrificing square footage. Thirdly, designers can consider alternate construction methods, such as prefabrication, that can result in greater material and construction efficiency.
Conclusions—a Call to Action
Accounting for total life cycle carbon and dramatically reducing the up-front and near-term emissions of buildings is imperative to meeting carbon reduction targets. As climate action emerges as a key mission common to building owners, institutions, and practitioners, we must creatively and collaboratively utilize all of the tools and data at our disposal to transform the way we design, renovate, and inhabit our built environment.
To truly move the needle, we must consider the full environmental costs of our work. Every project has a dollar budget, and decisions are made throughout a project to ensure that the budget is met. While carbon emissions are generally not yet associated with a financial cost, we are all working with a carbon budget, and tracking our carbon costs as closely as we track dollar costs is the next step towards meeting carbon reduction targets. The resources and tools we need are here and improving, and we must immediately reframe how we count carbon and dramatically rethink the way we do business to transform how we shape the built environment for a sustainable future.
—Lori Ferriss AIA, PE, LEED AP, is an associate at Goody Clancy and a founding member and chair of the Zero Net Carbon Collaboration for Existing and Historic Buildings (ZNCC).
- [i] UN Environment Gap Report 2018
- [ii] Architecture 2030, “50x30 or Bust!” August, 2019
- [iii] World Green Building Council, Global Status Report 2017
- [iv] UN Global Report 2017
- [v] IEA (2017), Tracking Clean Energy Progress 2017, IEA/OECD, Paris, iea.org/etp/tracking2017
- [vi] Architecture 2030, materialspalette.org/concrete
- [vii] Pak, Anthony, “Concrete High Rise LCA Study, City of Vancouver,” May 28, 2019.
- [viii] Magwood, Chris, “Opportunities for Carbon Dioxide Removal and Storage in Building Materials,” 2019.
- [ix] “Assessing the Carbon-Saving Value of Retrofitting versus Demolition and New Construction at the United Nations Headquarters,” December 6, 2016.
- [x] “The Total Carbon Study,” November 13, 2015